Understanding Battery Energy Density: Why It Powers the Future
Battery energy density (Wh/kg and Wh/L) continues to rise from lead-acid, lithium iron phosphate, NMC/NCA to solid-state battery levels, achieving longer battery life and lighter volume, but also exacerbating thermal runaway and raw material risks; in the future 2025-2030, solid-state electrolyte, lithium sulfur, sodium ion and silicon-based technologies will jointly optimize safety, cost and cycle life to meet the diverse needs of consumer electronics, smart travel and grid energy storage.
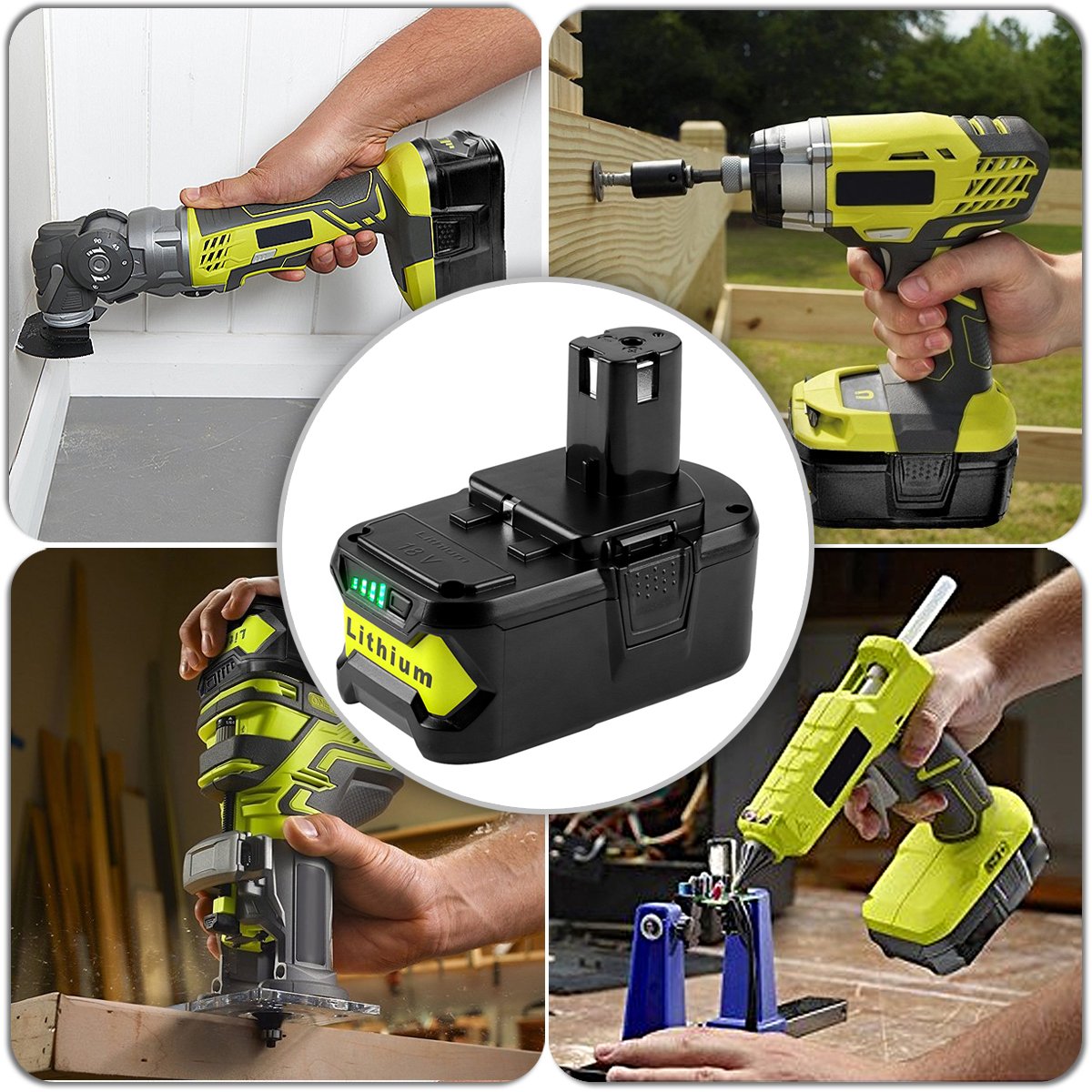
Battery energy density measures the amount of energy stored per unit of mass or volume (Wh/kg or Wh/L). Higher energy density enables longer runtimes, lighter weight, and more compact designs—fueling advancements in electric vehicles, portable electronics, and grid storage. As researchers push densities above 300 Wh/kg with next-generation lithium-metal and solid-state technologies, we can expect faster charging, extended range, and safer batteries, accelerating the shift to sustainable transportation and renewable-powered futures.
What Is Battery Energy Density?
Battery energy density quantifies how much electrical energy a battery can store relative to its weight (Wh/kg) or volume (Wh/L). Higher energy density means longer runtimes and lighter, more compact packs—critical for electric vehicles, drones, and portable electronics. For example, a 250 Wh/kg pack holds 250 watt-hours per kilogram, enabling greater range without extra mass. Advances in cell chemistry, like lithium-metal and solid-state designs, are steadily pushing these figures higher.
This section dives deep into the term “energy density,” distinguishing between gravimetric and volumetric measures:
- Gravimetric Density (Wh/kg): Energy stored per kilogram of battery mass. Critical when weight is limited (e.g., drones, EVs).
- Volumetric Density (Wh/L): Energy stored per liter of battery volume. Essential for devices where space is constrained (e.g., smartphones, grid racks).
- Not to Be Confused With: Specific power (W/kg), cycle life (number of charge/discharge cycles), or safety ratings.
Energy density is a primary design driver: higher Wh/kg or Wh/L means more energy in less mass or space.
Why Does Energy Density Matter?
Higher energy density means a battery stores more watt-hours in the same mass or volume, translating directly into longer runtimes, lighter weight and smaller pack size. This boosts electric vehicle range, extends tool run times and shrinks electronics without adding bulk. Greater energy density also reduces charging frequency and material use, lowering costs and environmental impact—making it a cornerstone metric for advancing portable power and sustainable transportation.
This section explains why energy density is critical for various end uses—smartphones, power tools, EVs, and grid storage—and how each application demands different aspects of density:
- Smartphones: Users want all-day runtime without adding bulk. High volumetric density enables slim form factors with large-capacity batteries.
- Electric Vehicles & Drones: Extended range is directly tied to gravimetric density. Every extra Wh/kg extends mileage or flight time without increasing payload.
- Grid Storage: High volumetric density reduces the physical footprint and civil works cost for each installed kWh.
Different scenarios balance weight, volume, cost, and safety considerations around density.
How Does Energy Density Vary by Battery Chemistry?
This section uses a comparison table of mainstream chemistries to highlight performance differences in both gravimetric and volumetric terms:
Chemistry | Gravimetric (Wh/kg) | Volumetric (Wh/L) | Typical Applications |
Lead-Acid | 30–50 | 80–90 | Backup power, automotive SLAs |
LFP (LiFePO₄) | 90–120 | 220–300 | EV buses, stationary storage |
NMC (LiNiMnCoO₂) | 150–220 | 450–650 | Consumer electronics, EVs |
NCA (LiNiCoAlO₂) | 200–260 | 600–800 | High-end EVs (e.g., Tesla) |
Solid-State | 280–500 | 700–1,200 | Next-gen EVs, aerospace prototypes |
Lab-scale prototypes reaching peak theoretical densities.
What Are the Advantages of High Energy Density?
This section focuses on three core advantages of pursuing higher Wh/kg and Wh/L:
1. Longer Runtime/Range: Devices can operate longer between charges, critical for portable electronics and electric vehicles.
2. Reduced Weight & Size: Less mass and volume for a given capacity—vital for drones, wearables, and compact devices.
3. Lower Packaging Costs: Higher density reduces the total number of cells and associated packaging, simplifying thermal management and lowering system costs.
High energy density accelerates innovation across mobility, consumer, and grid sectors.
What Are the Challenges and Risks of High Energy Density?
This section analyzes key bottlenecks and safety considerations when pushing energy density:
- Thermal Runaway & Safety Hazards: Higher energy stored in smaller space increases fire risk if abused or damaged.
- Materials Scarcity & Cost: Cobalt/nickel-based chemistries face supply constraints and high raw material prices.
- Faster Degradation Trade-offs: High-density cells often suffer accelerated capacity fade over cycles.
- Manufacturing Complexity: Tighter tolerances and advanced materials demand stringent quality control and drive up production costs.
Balancing energy density with safety, durability, and cost remains a major R&D focus.
What Are the Future Trends in Battery Energy Density Between 2025 and 2030?
This section looks ahead at emerging technologies and their density targets:
- Solid-State Electrolytes: Aiming for >350 Wh/kg by combining lithium-metal anodes with ceramic or polymer electrolytes.
- Lithium–Sulfur: Lab prototypes already achieve ~500 Wh/kg; commercial targets of 350–400 Wh/kg by 2030.
- Sodium–Ion Batteries: Lower theoretical density (120–160 Wh/kg) but very low cost and abundant materials—ideal for grid storage.
- Advanced Anodes & Recycling: Silicon, lithium-metal anodes, and closed-loop recycling techniques to recover critical metals and improve circularity.
Multiple parallel research paths promise to boost practical energy densities and lower system costs.
How to Choose Batteries Based on Energy Density for Different Applications?
Choose batteries by matching energy density to your application’s needs. For slim devices, prioritize high volumetric energy density (Wh/L). For drones and tools, select batteries with high gravimetric energy density (Wh/kg) and strong discharge rates. EVs need both high gravimetric and volumetric density for extended range. Grid storage values lifespan and safety over density. Always balance size, weight, and power demands for optimal performance.
This section provides a practical selection guide based on density profiles:
- Consumer Electronics: Prioritize high **volumetric density** (Wh/L) to minimize device thickness while maximizing capacity.
- EVs & Drones: Balance high **gravimetric density** (Wh/kg) with safety and cost—look for NMC/NCA or advanced solid-state cells.
- Grid Storage: Optimize **cost per Wh** over peak density—LFP or sodium-ion often deliver the best overall value for stationary use.
Match the density metric to the primary constraint—weight vs. space vs. budget.
FAQ
This section lists common reader questions with concise answers to clear up misconceptions:
Does Higher Energy Density Mean Shorter Life?
Not necessarily—cycle life depends on the interplay of chemistry, cell design, thermal management, and BMS algorithms.
What’s the practical “sweet spot” for EVs?
Currently around **200–250 Wh/kg**, balancing range, cost, and durability for mainstream electric vehicles.
Why Aren’t Solid-State Batteries Everywhere Yet?
Scale-up challenges in manufacturing, electrolyte stability issues, and high costs hinder mass commercialization.
What Are the Next Steps After Understanding Battery Energy Density?
This section summarizes key takeaways and directs readers to further resources and tools:
Empowered with this knowledge, you can make informed battery choices that drive innovation and sustainability.